How the NYU Genome Center is Making Better Cell Therapies
Neville Sanjana, PhD, creates tools to understand the impact of genetic changes on cancer evolution and to engineer new gene and cell therapies. He is a Core Faculty Member at the New York Genome Center. He also holds a joint appointment as Associate Professor in the Department of Biology at New York University and is an Associate Professor of Neuroscience and Physiology at the NYU School of Medicine.
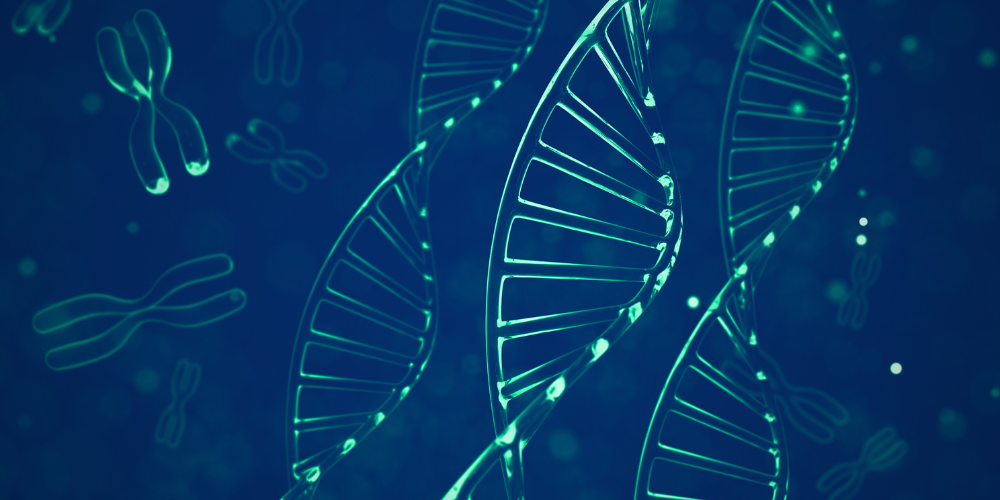
What work are you leading at the NY Genome Center and NYU?
We develop tools to better understand the human genome, through the ability to edit it. For the last few decades, we’ve been able to read the code of human genetics well, but it’s difficult to fundamentally understand something if you can’t manipulate it or edit it. We use genome editing tools to understand human genetics and cancer genetics.
How does your work understanding the genome apply to cancer immunotherapy?
Cell therapies, in which a patient's T cells are engineered to target tumor cells and eradicate them, are amazing breakthroughs for some blood cancers. But for most cancers, which are solid tumors, there are no effective or approved cell therapies. We're trying to understand why that is and what we can do to build better cell therapies.
To do this, we've developed large scale genetic approaches — where we can knock out or overexpress all 20,000 genes in the genome — and then ask which genes are relevant to the disease or drive a therapeutic response. But genes are only a tiny fraction of the genome. So, we’ve also used genome engineering to look at the other 98% of the genome that does not encode genes. These parts of the genome often serve as knobs and dials modifying where and how genes are expressed. By understanding the functional roles of different genes and different non-coding parts of the genome, the overall goal is to understand how we can make those engineered cell therapies better, and what genes help to enable that.
"One reason that CRISPR nucleases caught on throughout the biomedical research community is that they're very programmable."
What tools are you using to edit and understand cancer genetics?
I’ve worked with a few different genome engineering tools in my career – zinc finger nucleases, TALE nucleases, also known as TALENS, and of course, CRISPR. That is what we mostly focus on now. One reason that CRISPR nucleases caught on throughout the biomedical research community is that they're very programmable.
Instead of just targeting genes one at a time, we can quickly look at all 20,000 genes in the human genome, or regions outside of genes, and do it at scale. That lets us ask wide-ranging questions like, “What are all genes that contribute to tumor drug resistance?” “What are all genes that contribute to tumor growth or to metastasis?” That is what we do: Take advantage of new capabilities in genome editing and DNA synthesis to cast a wide net and find surprising discoveries that are often hidden in small corners of the human genome. It really is like being an explorer and makes every day in the lab full of surprises and new finds.
What are the main characteristics of T cells that you're most interested in understanding?
In our recent study, we asked, “What are all the genes that make T cells proliferate better?” If you look at CAR-T patients who have responded well to cell therapies and are now cancer-free, you can sample their blood and often find these engineered T cells still there. So not only did you cure them of the cancer, but you installed something like a permanent surveillance security system that is wandering around looking for any cancer recurrence and ready to take it out. And so if you can create something durable, that would be very impactful. Of course, all approved cell therapies today are autologous and getting high quality T cells from sick cancer patients is less than ideal. For an allogeneic cell therapy, where one cell donor can be used to treat many patients, T cell proliferation and persistence is a major problem and thus our screen suggests new ways to rejuvenate and prolong the life of those cells.
More generally, the tools that we've developed allow us to quickly search through the genome and to understand the function of different genes. So if you have a way to look at many genes quickly, and identify the most relevant ones to improve a particular aspect of T cell function, then it's all about picking the best function(s) to improve. If we can optimize several key properties of T cells, like persistence, trafficking to the tumor, preventing allo-rejection and resistance to exhaustion, then we can understand the key genes that are needed for an effective and durable cell therapy.
Why did you begin with proliferation?
For several reasons. First, proliferation is one of the easier things to measure and several studies have shown that more T cells result in quicker and more complete tumor remission. Second, when we talk about making cells proliferate, it's not just about proliferation in the body after they are created. It’s even earlier: at the time of manufacturing. During the pivotal trial that led to the approval of the first CAR-T, Kymriah from Novartis, for about 15% of patients, they weren't able to produce enough T cells at the manufacturing site to put back into the patients. Those patients didn’t even get the chance to try CAR-T therapy.
What did you learn about the characteristics of successful T cells?
That’s right. Not all T cells are equal. Some can be youthful with lots of potential to proliferate and kill tumor cells and some are exhausted or dysfunctional. For the youthful T cells, we can identify a set of T stem cell markers. For example, T cells with central memory stem markers have a longer life than an effector T cell, which is the most terminally differentiated type of T cell.
We found that a lot of the genes we discovered in our work in proliferation seemed to create that stem-like phenotype in the T cells. So if you can screen for that, you can select them. It’s similar to T cells being resistant to exhaustion: we know the importance of PD1, CTLA4, TIM3, or LAG3 – those are all markers of exhaustion. That could be another great characteristic to screen for. We screened for proliferation, but we found it improved those other areas as well.
How could your work optimize current therapies, or help to address newer challenges like durability in allogeneic products?
In our work, we overexpressed 12,000 genes – not quite every gene in the human genome, but a large number of them – and we looked to see which genes, when overexpressed, increased proliferation of T cells from diverse donors.
We showed in our research that these modifiers can be used for many different kinds of T cell therapies. We combined them with different FDA-approved CD19 CARs. We showed that they potentiated those responses of proliferation. There is also work using T cell receptors (TCRs), like NY-ESO-1, for melanoma, renal cell carcinoma, etc. We showed that when we put modifiers in that setting, it also worked. And when you put them in an allogeneic setting – we used γδ T cells in our case – these modifiers also worked. Many of the modifier genes that we found, including the #1 hit, a gene called LTBR, worked in all contexts.
"We felt there was an opportunity to tune T cells to make them stronger and more resistant, and find new genes that can be combined with any of those other strategies."
What does that tell us about cell therapies?
It is reassuring because it shows us that a T cell is a T cell no matter what. We need to create different therapies if you’re doing autologous, allogeneic, etc, but that these modifiers appear to work across many different T cell contexts.
In focusing on the T cell itself, rather than the antigen or the other parts of the CAR, how do you think your work impacts the broader field?
Very reasonably, a lot of the field is focused right now on finding new tumor antigens that are only found on tumors and not found on healthy tissues, or developing new receptors to bind to antigens, or a new kind of CAR or TCR that can recognize an antigen that is only found on tumor cells. This is an important and complementary goal.
Our question was if we could kick these cells into different states. We felt there was an opportunity to tune T cells to make them stronger and more resistant, and find new genes that can be combined with any of those other strategies.
The idea for us was to prove out this platform with a view to the clinic. We used the same delivery method as CARs, a lentivirus, and these modifier genes could be put into the same viral construct along with a CAR. For example, the Novartis product is a lentivirus that expresses the CD19 CAR, so we’re talking about just adding another gene next to it. In terms of manufacturing, our modified CARs can be made in exactly the same way as current FDA approved cell therapies.
CRISPR plays a huge role in your work. What has it elucidated around cancer mutations?
We've been able to understand the role of individual driver genes and how those drivers impact tumor physiology, metastasis, drug resistance, immunotherapy resistance and more. This is not a trivial challenge! Cancer genomes contain many, many mutations, so it can be tricky to pinpoint which ones are most relevant.
CRISPR gene editing has been very useful to separate out the true driver mutations in oncogenesis, from the passenger mutations that are present, but are not primarily responsible for cancer growth. This is even more true for mutations in parts of the genome that don't code for proteins, the 98% of the genome that's non-coding. It is much harder to understand the functional significance of any particular mutation in those regions.
Is CRISPR allowing you to experiment to understand the interplay of mutations in the cancer genome?
With CRISPR, we can actually mimic this in the lab. That is something we couldn't do before. Because of CRISPR, we can also make multiple mutations. We can choose the order of the mutations to compare different evolutionary paths to the same tumor genome. We can say which mutations are synergistic with each other.
And in the same way, we can find gene combinations that result in synthetic lethality. That is, if there is a certain mutation that drives cancer growth, are there other mutations that would not hurt healthy tissue but, because of the first mutation, are lethal to this tumor? This is great, because now we know that could be a targetable gene for a new drug target — and CRISPR screens have played a major role in identifying these kinds of targeted therapies.
For more information on IO360º Summit, click here.